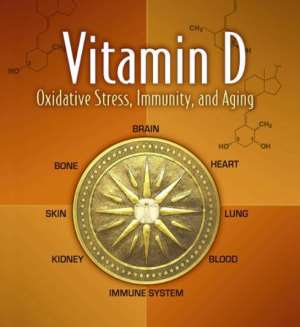
It is hypothesised, herein and suggested by others (Watkins, 2020), and in a paper by Grant and Lahore, (Grant et al., 2020) that vitamin D insufficiency may significantly compromise, respiratory immune response function, greatly increasing risk of COVID-19 ( Coronavirus ) severity and mortality.
This hypothesis focuses primarily on the contention vitamin D deficiency is the most critical factor in determining the extent, and severity, of COVID-19 infection, progression and outcomes. Further, it is not discussed in depth, but a matter of importance, that obesity reduces vitamin D in plasma, increasing risk of deficiency, and raising supplementation dosage requirements for correction of deficiency in the obese. “Serum 25OHD is inversely correlated with body weight, BMI and fat mass” and “about 20% lower in obese people than normal weight”. (Walsh, et al., 2017) Obesity and related comorbidities increase risk of poor outcomes in influenzas, thus likely the risk of COVID-19.
In respiratory system conditions, such as influenza, vitamin D has wide-ranging and fundamental roles, including through: gene transcription via COVID-19 relevant VDR (Vitamin D Receptor) pathways; wider immune function; and airway epithelial cell tight-junction function and integrity. Further, studies suggest vitamin D supplementation may be protective in respiratory conditions, the effect being highly significant in 'D' deficient persons.
The elderly, and those with pre-existing conditions, are at greatest risk. The article 'US studies offer clues to COVID-19 swift spread, severity' notes, “The first description of outcomes in 4,226 US COVID-19 cases reported to the CDC from Feb 12 to Mar 16 shows that 31% of cases, 45% of hospitalizations, 53% of intensive care unit (ICU) admissions, and 80% of deaths occurred in people 65 years or older.
Fatality rates for people 85 years and older ranged from 10% to 27%. Of those aged 65 to 84 years, 3% to 11% died. Death rates fell to 1% to 3% among those 55 to 64, less than 1% in those 20 to 54, and 0% in those 19 and younger.”
“Of the 2,449 infected patients whose age was known, 6% were 85 years or older, 26% were 65 to 84, 18% were 45 to 54, 17% were 55 to 64, and 20% were 20 to 44. Only 5% of infections occurred in people 19 years and younger.”(Van Beusekom, 2020) (Begley, 2020; Dong et al., 2020)
Mortality rates for COVID-19 differ significantly between countries, ranging from 8.3 % in Italy (20th March 2020) to between 0.27 and 1.0 % in northern European countries. The annual average 2011-2017 percentage mortality to infection rate of influenza in the USA is around 0.14 %.(Disease Burden of Influenza) The COVID-19 percentage mortality to infections rate is likely overstated, due to non-reporting of asymptomatic COVID-19. However, irrespective of the variation in mortality rate, COVID-19 is a new virus, with a capacity to spread rapidly.
Clearly it is important that the reasons for these differentials in mortality are better understood. Answers may provide ways to better manage the COVID-19 pandemic. Improved immune function, through better nutrition, is likely a factor. Nutrients such as vitamin D, and iodine, have important roles in cellular, including immune function. Less is known about iodine than vitamin D.
Of course, a range of nutrients, not just vitamin D are likely involved. Vitamin D co-factors include vitamin K2, vitamin A, magnesium and zinc. Interactions are complex. Insufficiencies of many nutrients, including vitamin D and iodine, are common place. Northern European diets are probably more nutrient dense than some, including due to consumption of whole grain minimally processed breads. Due to their culture and more northerly latitude, they tend to include greater amounts of vitamin D rich foods, and have greater focus on supplementation.
What constitutes vitamin D deficiency in adults is debated, but for the purposes of this hypothesis, is taken to be plasma levels < (less than) 25nmol/L (10ng/ml). This hypothesis focuses on vitamin D deficiency, as a likely key factor, but by no means only factor, in COVID-19 progression and outcome. Plasma vitamin D levels are inversely related to the amount of vitamin D ingested in food, and made in 'summer sun' months, following exposure to sun. Levels are reduced by obesity.
Vitamin D related immune pathways, have been demonstrated to be active in the progression of COVID-19, in a patient. Wider research suggests, vitamin D is protective against respiratory conditions. Population studies suggest that those with vitamin D deficiencies are more susceptible to respiratory illnesses, including influenzas.
COVID-19 clinical manifestation and time progression
The paper 'The outbreak of COVID-19' provides an overview of the COVID-19 virus clinical manifestation, and time progression; “COVID-19 has a mean incubation period of 5.2 days (95% confidence interval, 4.1–7.0). The infection is acute without any carrier status. Symptoms usually begin with nonspecific syndromes, including fever, dry cough, and fatigue. Multiple systems may be involved, including respiratory (cough, short of breath, sore throat, rhinorrhea, hemoptysis, and chest pain), gastrointestinal (diarrhea, nausea, and vomiting), musculoskeletal (muscle ache), and neurologic (headache or confusion). More common signs and symptoms are fever (83%–98%), cough (76%–82%), and short of breath (31%–55%). There were about 15% with fever, cough, and short of breath. Conjunctival injection was not reported in the early series and cases with age under 18 were few. After onset of illness, the symptoms are somehow mild and the median time to first hospital admission is 7.0 days (4.0–8.0). But the disease progresses to short of breath (~8 days), acute respiratory distress syndrome (ARDS) (~9 days), and to mechanical ventilation (~10.5 days) in about 39% patients. Patients with fatal disease develop ARDS and worsened in a short period of time and died of multiple organ failure. The mortality rate in the early series of hospitalized patients was 11%–15%, but the later statistics was 2%–3%.”(Wu et al., 2020)
Uncertainty as to extent of COVID-19 infection
The level of COVID-19 infection in populations is uncertain, due to; very mild even asymptomatic manifestation in some; inherent lags in availability of testing facilities; compounded by, social stigma attached to earlier infected persons, personal economic and social consequences; all magnified by historical lack of testing capacity in many countries. Additionally, a number of testing mechanism are being used, and until they are directly compared, there is no certainty measurements are equivalent.
Low COVID-19 death rate in Germany - hospital situation as at 20th March 2020
Germany as at 20th March 2020, has a comparatively low COVID-19 percentage death rate to infections; 0.34 % representing 68 mortalities, out of 19,848 reported infections. The German Government is nonetheless taking a reasonable and prudent position, in the face of uncertainty, and absence of adequate information as to the characteristic of COVID-19.
Consistent with the comparatively low death rate to date, a media source 'Speigel International' (Ker, 2020) reported quotes from Medical Professionals, suggest hospitals are not yet seeing a large influx of COVID-19 patients. Germany has significantly more acute bed capacity than Italy, but nonetheless, there are no media reports as of 19th March 2020, of significant pressures on hospitals
This suggests that data for Germany on mortality, and anecdotal suggestions of a lower impact of COVID-19 in Germany, are reasonable. Indeed, media asked why the death rate in Germany is low(Monella & Priese, 2020). Speigel International quotes include:
“But how quickly are hospitals responding to Spahn's (German Minister of Health) "urgent appeal"? Are procedures really being cancelled; are intensive care unit beds being freed up? "We had to cancel all operations on Monday because I had been in a risk area myself and hadn't been tested yet," a chief physician at one northern German hospital told DER SPIEGEL. "Apart from that, we haven't postponed any specific procedures yet, because there are no corona-infected patients in our hospital."(Ker, 2020)
“An anesthesiologist at a municipal children's hospital reported the situation is similar where he works. "Some parents have cancelled operations as a precaution," says the doctor, "a hernia or a gastroscopy usually doesn't have to take place immediately." He said things were pretty much business as usual at the hospital on Monday and that they were still performing outpatient operations. "Otherwise, we wouldn't have anything to do at the children's hospital right now," said the anesthetist.”(Ker, 2020)
“In terms of coronavirus patients, things are still quiet in many hospitals in Germany, and by no means will all facilities have to be hectically converted into emergency facilities. When contacted by DER SPIEGEL, the Schön-Kliniken, a company that operates 26 hospitals in Germany, said that clinics that don't have intensive care facilities are operating just as they have until now.”(Ker, 2020)
These reports do not suggest large number of patients in Germany are requiring intensive hospital treatment. Thus, the lower mortality effects are likely real, and not just reporting differences.
Similarly, Swedish COVID-19 data below, media reporting, and detail on the Wikipedia page, (Wikipedia contributors, 2020d) do not as yet, at 20th March 2020, suggest high levels of pressure on medical facilities, or 'large' number of seriously ill patients, albeit shortages of protective equipment were reported on 13th March 2020. Albeit there is less information on line, the situation appears broadly similar in other Nordic Countries.
COVID-19 progression and outcome by age group - CDC data
Recent data from the USA suggests adults in their mid-years account for a significant portion of infections, of which some are hospitalised. In the middle age group, the level of case severity of those hospitalised is not clear, but progressions to ICU of younger people is likely low. Current figures suggest risk of mortality in healthy persons in this age group is low.
The CDC report dated 18th March 2020 (Bialek et al., 2020), based on 2,249 patients, includes the table below (See Table 1).
Table 1. CDC COVID-19 data-table
“* Lower bound of range = number of persons hospitalized, admitted to ICU, or who died among total in age group; upper bound of range = number of persons hospitalized, admitted to ICU, or who died among total in age group with known hospitalization status, ICU admission status, or death.” Copied from the CDC web site with many and grateful thanks to the Authors.(Bialek et al., 2020)
COVID-19 data – vitamin D – infection and mortality rates
Whilst infection data is incomplete, there is clear mortality data; as well as observable more diffuse impact trends, including; hospital data, wider medical resource utilisation, and public pressure / media reporting trends, which together, likely broadly reflect the actual number of patients under treatment in each country.
As seen in table 2 below (See Table 2), there are striking differences between COVID-19 impacts in countries such as, China, Italy and France; and the northern European countries. Despite having a significant number of cases, and in some instances very similar reported infection progression profiles (as seen in France and Germany), the northern European countries currently consistently suffer much lower mortality to infection percentage rates (Figure 2).
Further, based on available limited data; a paucity of media suggestions that COVID-19, was at 20th March, causing actual significant consequential requirements for extra hospital facilities; general media coverage of public information announcements, and population responses; it appears the pandemic in northern European countries, is currently less impactful, than in southern European countries, notably Italy and Spain.
Lower vitamin D deficiency in northern Europe?
Whilst as noted, limited recent European data is available, the paper “Current vitamin D status in European and Middle East countries and strategies to prevent vitamin D deficiency: a position statement of the European Calcified Tissue Society”, observes, “Vitamin D deficiency (serum 25-hydroxyvitamin D (25(OH)D) <50 nmol/L or 20 ng/mL) is common in Europe and the Middle East. It occurs in <20% of the population in Northern Europe, in 30–60% in Western, Southern and Eastern Europe and up to 80% in Middle East countries.”(Lips et al., 2019)
A lower level of vitamin D deficiency in northern Europe, would be consistent with their National public health policies as to fortification, as well as promotion of the need for supplementation, particularly in the elderly, and understanding by a public; and again particularly the elderly, acquainted with issues of bone degradation; yearly faced with long dark nights, in northern latitudes; of the need for vitamin D supplementation.
Greater vitamin D deficiency in southern Europe
In contrast, to northern Europe, vitamin D levels in southern and eastern Europe may be both lower, and continuing to fall. The 2018 review, 'Vitamin D status among Mediterranean regions' observes “Southern European countries are at greater deficiency risk than Northern ones.” “A recent systematic review confirmed the high prevalence of low vitamin D concentration in countries of Southern Europe and Eastern Mediterranean regions. Whereas food fortification with vitamin D and vitamin D supplementation were common practice in northern Europe, in the Mediterranean regions were highly underestimated. Additionally, further research showed that sun-seeking behavior is rather avoided in southern countries, both due to heat avoidance or melanoma prevention guidelines.”(Kasapidou et al., 2018)
Figure 1. Plot of the total number and time-lines of COVID-19 infections and deaths in; Italy, France and Germany. The data was taken from a mixture of, WHO 'Situation reports', (WHO Coronavirus disease (COVID-2019) situation reports), Worldometer (“Coronavirus Update (Live): 274,171 Cases and 11,354 Deaths from COVID-19 Virus Outbreak - Worldometer,” n.d.), and Wikipedia (Wikipedia contributors, 2020b, 2020a, 2020c). (For German data, there appears to be a one-day difference between the data on the Wikipedia page and WHO figures, and those on Worldometer, hence the difference in German figures, between those in Fig.1, and Figs.2 and 3.)
“Another aspect could be the urbanization that is observed in all Southern European regions. Studies have shown that there is a higher prevalence rate of vitamin D insufficiency in urban populations due to the modern lifestyle and office jobs that entail to less time outdoors. Fortification policies and the overall capacity of the diet to supply enough vitamin D to maintain healthy serum 25-hydroxyvitamin D concentration (S-25(OH)D) failed to be as effective as expected.”(Kasapidou et al., 2018) .
Table 2#. COVID-19 infection cases, total death, serious/critical patients, vitamin D levels and percentage population with <25 nmol/L vitamin D levels, in different countries on 18th March 2020.
Vitamin D (nmol/L)a | Population with <25 nmol/L of Vitamin D (%)b | Total Casesb | Total Deathb | Serious/ Critical Casesb
| % Death to Infection | Vitamin D intake mcg elderly Male z | Vitamin D intake mcg elderly Femalez | |
Iceland | 57.0 | 4.2 | 409 | 0 | 1 | - | - | - |
Norway | 65.4 | 0.29 | 1959 | 7 | 27 | 0.36 | 15.0 | 12.9 |
Sweden | 72.9 | 0.8 | 1639 | 16 | 21 | 0.98 | 7.1 | 6.1 |
Finland | 67.7 | 0.2 | 450 | 0 | 2 | - | 9.0 | 6.5 |
Denmark | 65.0 | 0 | 1255 | 9 | 37 | 0.72 | 3.9 | 3.1 |
UK | 47.4 | 15.4 | 3983 | 177 | 20 | 4.44 | - | - |
Ireland | 56.4 | 11.1 | 683 | 3 | 6 | 0.44 | 3.5 | 2.2 |
Netherlands | 60.8 | 4.3 | 2994 | 106 | 210 | 3.54 | 4.8 | 3.6 |
Belgium | 49.3 | 7.3 | 2257 | 37 | 164 | 1.64 | - | - |
Germany | 50.1 | 4.2 | 19848 | 68 | 2 | 0.34 | 4.4 | 3.4 |
France | 60.0 | 6.3 | 12612 | 450 | 1297 | 3.58 | - | - |
Italy | 45.0 | 54.5 | 47021 | 4032 | 2655 | 8.58 | - | - |
Switzerland | 42.6 | 15.2 | 5615 | 56 | 141 | 1.00 | 2.5 | 2.4 |
Poland | 32.5 | 25.0 | 425 | 5 | 3 | 1.18 | - | - |
Iran | 36.3 | 30.4 | 19644 | 1433 | - | 7.29 | - | - |
Russia | 33 | 40.1 | 253 | 1 | - | 0.39 | - | - |
Spain | 42.9e | 47e,f, | 21571 | 1093 | 939 | 5.07 | 0.7 | 0.7 |
China | NA | 70.3c | 80967 | 3248 | 2136 | 4.01 | - | - |
South Korea | NA | 70.2d | 8652 | 94 | 59 | 1.09 | - | - |
USA | NA | 30.6c | 19383 | 256 | 64 | 1.32 | - | - |
a The values of Vitamin D, and population numbers with <25 nmol/L of Vitamin D (%) (except for China, Spain, South Korea and USA) have been taken from reference (Lips et al., 2019)
b The values of total cases of COVID-19; infection; death; serious and critical patients; have been taken from reference (Coronavirus Update (Live): (“Coronavirus Update (Live): 274,171 Cases and 11,354 Deaths from COVID-19 Virus Outbreak - Worldometer, n.d.) on 20 March 2020. Figures vary sligthly between sources; for example between the WHO and Worldometer, thus figures are offered as indicational only – but in general direction terms, are consistent.
c The values of population with <25 nmol/L of Vitamin D (%) for China and USA have been taken from reference (Wei et al., 2019)
d The value of population with <25 nmol/L of Vitamin D (%) for South Korea has been taken from reference (Park et al., 2018)
e Spain was included in the (Lips et al. study), but no data was included on the Spain data line. Many of the studies in Europe used in (Lips et al. study) were 2015 and 2016, hence the selection of this study and the data it used for this group. The authors of this hypothesis, have also found difficulty in finding relevant data for Spain. (Spiro, 2014) data for an elderly group has been used for nmol/L. The other data was not used as two genders were specified.
f A Spanish study (Peláez V, 2017) indicates higher mortality in patients with low vitamin D under 12.48 nmol/L. 74 institutionalised patients had a mean level of 18.40 ± 7.58 nmol/L. The study makes reference to (Portela, 2009), a very small which found 47 % of institutionalised women from Llieda, age was over 65, were <25nmol/L. The 47 % figure is used as indicational only. Inclusion of the (Peláez V, 2017) nursing home data would have substantially raised the % <25nmol/L.
# Adult population was considered for all values of vitamin D levels and percentage population with <25 nmol/L of Vitamin D with age of >17 years (except UK). Due to unavailability of corresponding data the values for age group 1.5 to 91 years have been considered.
z Male and female elderly group vitamin D intake; data from their Table 10, from reference (Spiro & Buttriss)
'Residential' institutions – high vitamin D deficiency risk environments
The extent of vitamin D insufficiency is magnified in those residing in institutions; with low access to sunshine; often subject to nutritionally limited diets; and likely generally not having the benefits of organised supplementation regimes; such as; prisons,(Nwosu et al., 2014; Prisoners have very low vitamin D and get TB, influenza, and depression. n.d.) retirement homes (including those in Sweden),(Arnljots et al., 2017) mental institutions,(Cuomo et al., 2019) long stay hospitals, and submarines. Numerous studies confirm those in institutions are at risk of low Vitamin D levels.
Very sadly the greater susceptibility of those in residential care has been illustrated by events. In respect of COVID-19, the CIDRAP commentary article observes “The report on the coronavirus outbreak in a King County, Washington, nursing facility, which sickened 129 people (81 of about 130 residents, 34 of 170 workers, and 14 visitors) and killed 23 from Feb 27 to Mar 9, underscores the ability of the novel coronavirus to spread quickly in such settings.”(Van Beusekom, 2020)
The article continues “Of those infected, the median age was 81 years (range, 54 to 100) in residents, 42.5 years in staff members (range, 22 to 79), and 62.5 years (range, 52 to 88) for visitors. Eighty-four (65.1%) were women. (Van Beusekom, 2020)
Hospitalization rates were 56.8% for residents, 35.7% for visitors, and 5.9% of staff members. Death rates were 27.2% for residents and 7.1% for visitors; no staff members died. The most common underlying diseases in residents were high blood pressure (69.1%), heart disease (56.8%), kidney disease (43.2%), diabetes (37%), obesity (33.3%), and lung disease (32.1%). The only underlying condition in six residents and one visitor was high blood pressure.” (Van Beusekom, 2020)
If such high hospitalisation rates and mortality are common in residential institutions, ensuring COVID-19 protective factors are optimised, including resolution of vitamin D deficiency, if determined by studies to be relevant, could yield significant health and resource dividends.
Vitamin D deficiency: a factor in COVID-19?
Whilst currently there is insufficient data, and evidence, to come to any conclusion as to; the impact of vitamin D on; COVID-19, infection, hospitalisation or mortality rate; consideration from a wide perspective, including: known COVID-19 immunity pathways; immune pathways; vitamin D physiology; population studies on vitamin D levels and related respiratory condition outcomes; vitamin D clinical studies; and wider information; suggests vitamin D deficiency is likely to prove to be, a significant factor in COVID-19 progression and risks.
Interestingly, in figure 2, below, the total COVID-19 infection rates in different countries, are seen to be:
- correlated, to an extent, with: the percentage of vitamin D deficiency in the population - those with less than 25 nmol/L Vitamin D level,
- and inversely correlated, to an extent, with: both; higher plasma vitamin D levels, and, higher dietary vitamin D intakes.
It is noticeable, that, the level of COVID-19; infections, serious/critical cases, and mortality rates; are significantly lower; vitamin D intake higher; plasma vitamin D nmol/L higher; and vitamin D deficiency <25nmol/L lower; in Nordic and northern European countries.
Vitamin D data Caveat - graphical indications of National vitamin D status
As discussed, there is a paucity of recent population data on vitamin D. Much of it is old. Dietary habits (avoidance of dairy, specialist diets, movements towards vegetarian and vegan diets, reduction of intake of offal, etc.); time spent indoors; avoidance of sun; greater use of sunscreens; all factors impacting vitamin D status; have changed considerably in recent years.
Dietary data, is in any event, inherently difficult to quantify as set out in (Lips et al., 2019) and (Spiro, 2014), which were the two primary data sources used. The review, (Lips et al., 2019), in respect of several European countries helpfully cites comparatively 'recent' data from 2013-2017, but which is still some years old, a reflection of the lack of recent data. Changes in attitudes to supplementation since then could help account for differentials.
Thus, data graphed below, should only be taken as indicational of intakes in northern, and southern Europe, and more widely. There is a consensus in these two reviews, that vitamin D intakes, and blood parameters, are indeed higher, in northern than southern Europe, but for exact quantification, including consideration of relevance to COVID-19, data from new sepcific studies is requried.
Figure 2. Graphical representation of; total COVID-19 deaths, and serious / critical cases at 20th March, 2020, plotted against: vitamin serum 25OHD3 levels (nmol/L); % number of persons deficient in vitamin D <25 nmol/L Vitamin 25OHD; across a range of countries; based on data sources and studies as listed in Table 1.
Physiological importance of vitamin D in respiratory and wider conditions
In modern human biology, vitamin D along with vitamin A, and oxidised lipids of Omega 3 and 6, are signalling factors within a “superfamily of transacting transcriptional regulatory factors”,(Sertznig et al., 2009) which includes the vitamin D receptor (VDR).
Vitamin D, as well as factoring, including through gene transcription, in reproductive biology,(Keane et al., 2017) brain development; bone formation; and cancer; has roles in immune and inflammatory systems;(Kawahito, 2003) and of particular relevance to COVID-19, has significant roles in respiratory function.
Figure 3. Graphical representation of total infections, and total deaths at 20th March 2020; plotted against: vitmain D intake in mcg/day in elderly males and females; and the percentage of a wider population deficient in vitamin D; <25 nmol/L 25OHD; across a range of countries; based on data sources and studies as listed in Table 1 of this paper. Daily vitamin D intake is inversely related to; vitamin D deficiency, and % of blood levels of less than <25nmol/L. Data was taken from the British Nutrition Foundation (Spiro 2014) - their Table 10. The elderly data was selected as representing a group particularly at risk. The Spiro review sets out relevant caveats in the paragraph accompanying their Table 10. Whilst it is clear the Nordic countries have higher vitamin D intake; it is inherent in population dietary data that it can only be viewed as indicative.
The paper 'Genomic Determinants of Vitamin D-Regulated Gene Expression' observed, “From a genome wide perspective” “between 2000 and 8000 VDR-binding sites are detected following activation by 1,25(OH)2D3”.(Pike et al., 2016) The paper 'Vitamin D Effects on Lung Immunity and Respiratory Diseases,' observed “Vitamin D insufficiency has been linked to increased risk of infections, in particular viral respiratory tract infections”. 'Vitamin D and respiratory health'(Hughes & Norton, 2009) notes, “Vitamin D regulates more than 200 genes including genes for cellular proliferation differentiation and apoptosis”.
More specifically, a study mapping gene expression changes in a SARS-CoV model, noted “The transcription factors identified in these studies (vitamin D receptor [VDR], cyclic AMP receptor binding protein 1 [CREB1], Oct3/4, hypoxia-inducible factor α2 [HIFα2]-Epas, p53, and SMAD4) play important roles in the regulation of a variety of cellular processes, including transforming growth factor beta induction, maintenance of normal lung cell functions, prevention of lung disease phenotypes, and proper immune cell functions”.(Sims et al., 2013)
The paper 'Epidemic influenza and vitamin D' lists other mechanisms by which vitamin D modulates immune response; “vitamin D, 1,25(OH)2D, a steroid hormone, has profound effects on human immunity. 1,25(OH)2D acts as an immune system modulator, preventing excessive expression of inflammatory cytokines and increasing the 'oxidative burst' potential of macrophages. Perhaps most importantly, it dramatically stimulates the expression of potent anti-microbial peptides, which exist in neutrophils, monocytes, natural killer cells, and in epithelial cells lining the respiratory tract where they play a major role in protecting the lung from infection.”(Cannell et al., 2006)
More widely, and thought provokingly, low vitamin D among 339 older adults with acute medical conditions, was associated with a higher risk of mortality in a hospital in France, in the winter season, January to October 2009.(Annweiler et al., 2010)
Pathways are further considered in the papers, 'Vitamin D and Influenza—Prevention or Therapy?'(Gruber-Bzura, 2018), 'An update on vitamin D and human immunity',(Hewison, 2012) and 'Role of Fat-Soluble Vitamins A and D in the Pathogenesis of Influenza: A New Perspective'.(Mawson, 2013).
Airway and lung function
The paper 'Vitamin D Effects on Lung Immunity and Respiratory Diseases'(Hansdottir & Monick, 2011) comments, “epidemiological studies have consistently found an association between low vitamin D levels and increased susceptibility to respiratory infections” and further notes, “localized synthesis of 1,25D rather than systemic production is responsible for many of the immune effects of vitamin D.”; it takes place in the airways (See Table 1 of Cited Paper).
The paper 'Vitamin D and respiratory health',(Hughes & Norton, 2009) observes, “a recent large cross-sectional study of the US population reported that vitamin D status is associated inversely with recent URTI (Upper Respiratory Tract Infections)”. Vitamin D is active in a wide range of immune systems; cytokines, lymphocytes, macrophages T cells, T helper cells; and related pathways IL-1, -2, -4, -5, -10, -12; Th1, -17, IFN-beta, CD14.
There are a large number of studies suggesting vitamin D plays important roles in, and may be protective against; asthma,(Martineau et al., 2016) acute respiratory infections including Influenza, (meta-analysis 10,000+ patients - those who were vit D deficient - below 25nmol/l – with improvement of vitamin D status, experienced a 70 % benefit – many globally are deficient – if this level of improvement in deficient patients was relevant to COVID-19, it would be highly significant)(Campbell, 2020; Martineau et al., 2017) COPD,(Jolliffe et al., 2019) bronchitis(Martineau et al., 2017) and tuberculosis.
Importantly, as set out in the review 'Treating the host response to emerging virus diseases: lessons learned from sepsis, pneumonia, influenza and Ebola', “There is growing recognition that endothelial dysfunction and the loss of endothelial barrier integrity are central to the pathophysiology of bacterial sepsis and acute lung injury”, ”Many systemic virus diseases, including influenza, dengue and Hantavirus pulmonary syndrome, are also characterized by endothelial dysfunction.” “The disruption of tight junctions between endothelial cells leads to a loss of barrier integrity, followed by the leak of fluid from the blood into interstitial tissues and beyond (e.g., the alveoli in pneumonia).” (Fedson D, 2016)
Crucially, vitamin D is essential to tight cell junction function, in the epithelial cells that line the lungs, and other such tissues including the gut. Interestingly, iodine and selenium, “alone, or in combination are able to strengthen the function of TJ's in human endothelial cells”.(Martin et al., 2005, 2007) The tight junctions help control passage of substances across the tissue barriers,(Zhang et al., 2013) between the external and internal space, including of fluids. Further, and of likely significance, 'Vitamin D Receptor Deletion Leads to the Destruction of Tight and Adherens Junctions in Lungs'(Chen et al., 2018).
An assayed immune pathway response in a COVID-19 patient
A report mapping a COVID-19 patient's immune response to COVID-19, in Nature Medicine, titled 'Breadth of concomitant immune responses prior to patient recovery: a case report of non-severe COVID-19', notes, “Since co-expression of CD38 and HLA-DR is the key phenotype of the activation of CD8+ T cells in response to viral infections, we analyzed co-expression of CD38 and HLA-DR. As per reports for Ebola and influenza, co-expression of CD38 and HLA-DR on CD8+ T cells (assessed as the frequency of CD38+HLA-DR+ CD8+ T cells) rapidly increased in this patient from day 7 (3.57%) to day 8 (5.32%) and day 9 (11.8%), then decreased at day 20 (7.05%).”(Galey & Hood, 2020)(Thevarajan et al., 2020)
Whilst research is diffuse, it is clear from other immune research, the CD38, HLA-DR, CD8, CD4 pathways have links to the VDR receptor, and thus are likely impacted by vitamin D deficiency. CD38 is a glycoprotein expressed on immune cells, which has significant roles in the innate and programmed immune function. Interestingly, the paper 'Relevance of Vitamin D Receptor Target Genes for Monitoring the Vitamin D Responsiveness of Primary Human Cells Of 12 target genes'(Vukić et al., 2015) notes, “in PBMCs (Peripheral Blood Mononuclear Cell – “a diverse mixture of highly specialized immune cells”(Muir, n.d.)) only the expression of the genes CD38 and TMEM37 kept a positive correlation with changes in 25(OH)D3 levels.”
VDR is linked to CD38. VDR agonist inecalcitol in melanoma cells, resulted in “5-fold increase in CD38 antigen density at the surface of the cells”.(Hybrigenics presented at the ASH Annual Meeting the potency of inecalcitol to induce CD38 on Multiple Myeloma cell lines, 2017) VDRs also have roles in control of CD4 and CD8 (surface glycoproteins). (Chen et al., 2014) “When guinea pigs were supplemented with 1,25(OH)2D3, the percentage of CD4+ and CD8+ T lymphocyte were significantly elevated and simultaneously, CD4+/CD8+ was reduced”.(Wang et al., 2017) HLA-DR surface receptor polymorphisms have also been associated in various studies with VDR.
Thus, vitamin D may well impact immune response to COVID-19 through: CD38, HLA-DR, CD8 and CD4; pathways identified in a patient, as highly relevant to COVID-19, infection progression, and resolution.
Mitochondria, immune function, and vitamin D
Mitochondria are truly central to cellular function. Vitamin D plays important roles in mitochondrial regulation and function, including in “redox homeostasis and protection against oxidative stress,”.(Santos et al. 2017) Thus, “Vitamin D is one of the key controllers of systemic inflammation, oxidative stress and mitochondrial respiratory function,”(Wimalawansa, 2019).
Mitochondrial energetics, and management of inflammatory oxidative stress, including apoptosis, (Vringer et al, 2019) are key determinants of outcomes in severe clinical conditions, including of tissue health. The paper 'Vitamin D Receptor Is Necessary for Mitochondrial Function and Cell Health' notes “Our data reveal that VDR plays a central role in protecting cells from excessive respiration and production of ROS that leads to cell damage.”, “ we conclude that VDR is essential for the health of human tissues.”(Ricca et al. 2018)
The review 'The Role of Vitamin D in the Immune System as a Pro-survival Molecule' observes, “The ability of vitamin D to check and mediate the biology of calcium as a signal molecule, however, relates its function to energetics (a task particularly associated with mitochondria and ER) and to the inflammasome(and immunity), just at the cellular level.” . . . “The overall amount of evidence reporting the anti-inflammatory and immunoregulatory action of vitamin D, in its active vitamin D3 forms, suggests a function that might be generally called “stress-quenching activity.” The ability of vitamin D to inhibit metabolic stress and energetic expenditure in a cell microenvironment and in contexts such as mitochondria or brown adipose tissue is intriguing. This ability suggests the existence of a wider task beyond its immune-tolerant or immune/anti-inflammatory role”.(Chirumbolo, 2017)
The ACE2 pathway may factor in regulation of mitochondria, including related endoplasmic reticular stress (Cao Xi).
Interaction of vitamin D receptor pathways with ACE, ACE2
ACE, ACE2, and related pathways, have been suggested to be implicated in COVID-19, an influenza like disease, which, when severe, results in lung damage evidenced by very fast shallow breathing, ending in terminal loss of lung function. A doctor described a COVID-19 patient “gasping for breath with every ounce of life that he could muster” “I could see the terror in his eyes. He knew.” (A Frontline Doctor for the Mail on Sunday, 2020)
A BMJ 'Preventing a COVID-19 pandemic' thread on COVID-19, contains the observation “SARS-CoV-2 uses ACE2 for target cell entry by fixing on it via its viral spike glycoprotein”, “SARS-CoV-2 downregulates ACE2”; it is postulated therein that upregulation of ACE2 may mitigate effects of COVID-19 (BMJ Preventing a COVID-19 pandemic - Responses. (Michely D.)
These pathways have been recognised as being of significant importance for the treatment of emerging viral diseases, as set out in the paper “Treating the host response to emerging virus diseases: lessons learned from sepsis, pneumonia, influenza and Ebola” which observes inter alia “Studies published more than a decade ago showed that ACE2 is the functional receptor for SARS coronavirus. Soon thereafter, ACE2 was shown to protect mice from acute lung injury (ALI) associated with experimental sepsis. This study suggested a broad role for ACE2 signaling in the pathogenesis of sepsis, acute lung injury and other forms of acute critical illness.”(Fedson D, 2017)
The paper 'VDR attenuates acute lung injury by blocking Ang-2-Tie-2 pathway and renin-angiotensin system.' observes “Taken together, these observations provide evidence that the vitamin D-VDR signaling prevents lung injury by blocking the Ang-2-Tie-2-MLC kinase cascade and the renin-angiotensin system.” (Kong J, 2013))
Acute lung injury ALI was induced in Wisestar rats using lipopolysaccharide, and calcitriol, a vitamin D3 'active form' analogue, was administered, “To observe the effect of vitamin D on angiotensin converting enzyme 2 ( ACE2 ) and vitamin D receptor ( VDR ) expression”. “The clinical manifestations (rapid shallow breathing; listlessness; the oral and nose hemorrhage) in LPS group were obvious, and the clinical manifestations and pathological changes of lung tissues in the LPS + calcitriol groups were significantly milder than those in LPS group.” The paper concluded “Calcitriol can increase the expressions of VDR mRNA and ACE2 mRNA and protein levels of VDR and ACE2 in rat models of LPS-induced ALI, thus suggesting the increased expressions of ACE2 mRNA and VDR mRNA playing a role in protection against the development of ALI.” (Yang et al. 2016)
A later study titled 'Vitamin D alleviates lipopolysaccharide‑induced acute lung injury via regulation of the renin‑angiotensin system' observed “Results from reverse transcription‑quantitative polymerase chain reaction, western blotting and ELISA analysis demonstrated that calcitriol also modulated the expression of members of the renin‑angiotensin system (RAS), including angiotensin (Ang) I‑converting enzymes (ACE and ACE2), renin and Ang II, which indicates that calcitriol may exert protective effects on LPS‑induced lung injury, at least partially, by regulating the balance between the expression of members of the RAS.” (Xu J 2017)
Thus, vitamin D deficiency may figure in the determination of the severity of COVID-19, and consequently, vitamin D; and or the active form, or an analogue such as 'calcitriol', in severe urgent cases; potentially, may be capable of being used in the clinical setting, for treatment purposes, in those with COVID-19 related respiratory conditions, to, as in the rodents above “improve clinical manifestations”. Arguably the necessary population and clinical studies should be undertaken as a matter of urgency, to determine these questions.
Global vitamin D deficiency
Multiple studies, from numerous countries, over the years, indicate that vitamin D deficiency and insufficiency, is a global issue.(Edwards et al., 2020; Roth et al., 2018) Reasons for rising plasma vitamin D deficiency, are multiple, and include; lifestyle changes resulting in less time outdoors, increased use of UVB blockers, desire for paler tone wrinkle free skin, smog blocking UV, fear of skin cancers, obesity, and often a lack of understanding of the wider importance of vitamin D by, Governments, many Doctors, Dentists, and the Public.
In consequence, population vitamin D levels are often falling, as seen in southern Europe, and even in more southerly latitudes; for example, it was reported in 2018, in Korea, “vitamin D status in South Koreans is still deteriorating” (Park, 2018)
Vitamin D levels vary between populations, which logically will have public specific, regional, health-effects. Providing a comprehensive 2020 global overview of vitamin D levels is not possible, as the data simply does not exist. Data examples are included in table 3 (See Table 3).
Table 3. Variation of vitamin D levels in different populations
Location | <10 or 12 ng Deficiency | <20 ng Insufficiency | Report |
Iran and Jordan | 50 % | 90 % | Arabi et al (2010) |
China | ~ 37 % | ~ 72 % | Zhang et al (2013) |
African continent | 18 % | 34 % | Mogire et al (2019) |
USA | 5 % | 18 % | Herrick et al (2019) |
Data with thanks from table in 'Comment' 'Vitamin D status in Africa is worse than in other continents'(Bouillon, 2020)
(To convert ng/ml to nmol/L multiply the ng/mL by 2.5 :- 10 ng/ml is equivalent to 25 nmol/L.)
Lack of current extensive data
Significant, meaningful, comprehensive, regular, population based public vitamin D data, will not exist until the importance of vitamin D to health is more widely recognised. There is no mechanism for global collection and reporting of anonymised individual medical vitamin D test data. In so far as testing takes place, vitamin D testing, even in vulnerable groups, in many countries, is not a priority.
This hypothesis calls for change, in vitamin D testing frequency, and national public health data collection, including wider recognition of the likely role of vitamin D in COVID-19, and wider health issues. Influenza Seasonal Trends – vitamin D a key factor?
Historically, between 1964 and 1975, before the advent of modern sun-creams, mass electronics, and fear of moderate sun exposure, there was a strong seasonal trend to increased winter influenza occurrence.(Cannell et al., 2006) This may logically have been associated with the seasonally changing, UVB related, vitamin D status of populations.
In more northern latitudes, the UV element of light is only sufficiently strong, to make vitamin D in exposed skin, during the summer months; the exact time frame is dependent on the latitude of the location.
Interestingly the paper 'Epidemic influenza and vitamin D' observes, “Volunteers inoculated with live attenuated influenza virus are more likely to develop fever and serological evidence of an immune response in the winter.”(Cannell et al., 2006)
The paper further notes, “Ultraviolet radiation (either from artificial sources or from sunlight) reduces the incidence of viral respiratory infections, as does cod liver oil (which contains vitamin D).” Vitamin D deficiency predisposes children to respiratory infections.”(Cannell et al., 2006)
It is also possible, increased ultraviolet light, and raised surface temperature due to light absorption heating effects, reduces life-span of the virus on clothes, and other surfaces, exposed to sun-light. In more recent times, use of UVB blocking sun-creams, and lifestyles that are focused to a greater extent on indoor activities, by reducing vitamin D status, may reduce the historical seasonality effect.
Possibility of seasonal re-emergence – warnings from history
Of possible relevance to COVID-19, concerningly, the history of Hong Kong flu, suggests seasonal effects may also be applicable to new flu variants. The paper 'Epidemic influenza and vitamin D' observes “For example, Miller et al. reported that the Hong Kong virus was first isolated in Britain in August 1968 but it did not cause significant summertime illness despite being a new antigenic variant in a non-immune population. However, clinical case rates increased in intensity as the sun became progressively lower in the sky each day (autumn), waiting until the winter solstice of 1968 before the first community outbreaks appeared. Influenza case rates peaked for several months but waned as the sun rose higher in the sky each day (spring). Predictably, influenza virtually ceased following the summer solstice. Clinical case rates for Hong Kong influenza (again) increased from September 1969, only to explode again in the days preceding the winter solstice, even though a much higher proportion of the British population had virus-specific antibodies at the beginning of the lethal second wave than they did at the beginning of its less lethal first wave.”(Cannell et al., 2006)
The then new, Hong Kong virus, did not manifest in summer, despite then access of the then new virus to a population with no immunity; given the historical seasonal trend of many influenzas, will COVID-19 follow this pattern? Further, and thought provokingly, the virus remerged more strongly the following winter; weekly influenza related consultations for diagnosed illness rates per 100,000, increased from 400 in February March 1969, to 1200 in December January 1970. See (Fig. 2 of cited study).(Cannell et al., 2006)
Clearly development and administration of a successful vaccine, may moderate the impact of any future reoccurrence of COVID-19 in 2021, but the elderly will remain particularly at risk. IF reduction of vitamin D deficiency, is shown to be protective against COVID-19, public policy to ensure populations were vitamin D sufficient, may help inhibit likely mid-year increase of COVID-19 in the Southern Hemisphere, or return to the Northern Hemisphere in winter 2021. Vitamin D and (25 hydroxy D)
Professor Holick, an acknowledged leader in the vitamin D field, provides an overview, in 'Vitamin D deficiency: a worldwide problem with health consequences'.(Holick & Chen, 2008) Humans in a preindustrial setting, mainly acquired vitamin D through the oxidation in the skin, by UVB, (but not UVA or longer light) of a cholesterol derivative to cholecalciferol, and to a lesser extent from dietary sources.
Vitamin D is stored long term in adipose tissue, and supplied for more current use, by serum to cells. Within cells, vitamin D is converted as needed to 25-hydroxyvitaminD3, also called D 25(OH)D3. This is further converted to the 1,25-D3 form ('calcitriol' is an analogue) (a VDR receptor agonist), and other metabolites.
The action of vitamin and its metabolites, is more complex than generally appreciated, impacting a range of genes.(Hassan-Smith et al., 2017) Conversion of vitamin D to metabolites happens mainly in the liver, but many cells possess conversion capacity, including as part of their immune function systems.
Importance – of vitamin D - a quasi-hormone - an evolutionary perspective
The wide biological importance of vitamin D, beyond its role in bone health, is often underappreciated, including in the Medical and Dental professions. Cholesterol like substances, were likely early evolutionary membrane building blocks, that helped facilitate light initiated oxidative stress messaging systems, and have been genetically conserved as integral factors in many biological structures, processes, and pathways.
The capacity of cholesterol, and other ring based organic molecules, to react with UV, and particularly UVB, gave them early relevance. In an early low atmospheric oxygen world; life forms needed mechanisms to protect their light exposed membranes from UV oxidation, and to provide them, with mechanisms to sense and respond to damaging UV light.(Brown, 2016b)
Further, on an evolutionary basis, cholesterol oxidised derivatives, exposed to both UV and solutions containing high levels of calcium, may have interacted with calcium ions in solution,(Marfey et al., 1975) leading to accumulation; helping provide mechanisms for calcification at surfaces. Interestingly, oxidised lipids and cholesterol are associated with calcium accretion in vascular systems.(Parhami et al., 1997; Tintut et al., 2018)
The likely early role of oxidised cholesterol like molecules, in external membranes, may explain the ongoing importance of an oxidised derivative of cholesterol, in regulation of tight cell junction function, and protection of barrier tissues from external threat, thus in immune systems. Similarly, the UVB oxidised products of linoleic acid retain central roles in immune biology, including as pain messengers.(Brown, 2016b)
Oxidised cholesterol remains relevant to external membrane function; indeed, a cholesterol derivative is oxidised to vitamin D on exposure to UVB, in the external and surface layers of the skin. As part of that process it retains a role in signalling external conditions, protecting against oxidative stress, and moderating behaviour to optimise function in relation to external UV stimulus.
Capacity to make Vitamin D
Possibly 90 %, of the vitamin D in plasma, of those with adequate vitamin D levels - who do not supplement; or eat fish, offal, eggs, dairy products, or sun exposed mushrooms; and do not eat processed vitamin D supplemented foods - is derived from the oxidative action of UVB, in summer strength sunlight, on a cholesterol derivative delivered to the skin. If they do not have sufficient UVB-skin-product-blocker-free, summer sun, exposure; they will be vitamin D deficient – it is almost impossible for them to be otherwise.
A light skinned Caucasian, who has not been exposed to the sun for a while, can make in the order of 20000IU of vitamin D. Dietary sources do not supply the same quantities, providing in comparison, limited amounts of vitamin D, as discussed below. Capacity of individuals to make vitamin D on exposure to sunlight is governed by a number of factors, including:
Exposure to UVB
Ethnicity and skin colour
Genetic polymorphisms
Age
Gender
Obesity
Regular use soaps etc.
Dietary sources
As noted by the National Institute of Health in the USA, natural food sources of vitamin D are very limited, and the amounts contained generally small.(Vitamin D — Health Professional Fact Sheet, n.d.) Fortified foods are the next best source of vitamin D. The range of foods fortified varies by countries, and level of fortification in individual foods is limited, including by government recommendation.
The USA NIH web advisory observes “Very few foods in nature contain vitamin D. The flesh of fatty fish (such as salmon, tuna, and mackerel) and fish liver oils are among the best sources. Small amounts of vitamin D are found in beef liver, cheese, and egg yolks. Vitamin D in these foods is primarily in the form of vitamin D3 and its metabolite 25(OH)D3. Some mushrooms provide vitamin D2 in variable amounts. Mushrooms with enhanced levels of vitamin D2 from being exposed to ultraviolet light under controlled conditions are also available.”(Vitamin D — Health Professional Fact Sheet, n.d.)
Many do not have access to oily fish, or do not eat it for fear of contaminants. Significant numbers have diets, which may exclude eggs meat and or dairy. (A serving of salmon contains between 400-800IU depending if it is wild or farmed - other fish contain much less – a bowl of fortified cereals may contain more). Without access to fortification, and or suitable sun exposure, people are likely to be deficient.
Fortification
For the reasons discussed above, fortification, and or supplementation, is essential for the avoidance of vitamin D deficiency, in many population groups. The NIH continues, “Fortified foods provide most of the vitamin D in the American diet. For example, almost all of the U.S. milk supply is voluntarily fortified with 100 IU/cup. (In Canada, milk is fortified by law with 35–40 IU/100 mL, as is margarine at ≥530 IU/100 g.) In the 1930s, a milk fortification program was implemented in the United States to combat rickets, then a major public health problem. Other dairy products made from milk, such as cheese and ice cream, are generally not fortified. Ready-to-eat breakfast cereals often contain added vitamin D, as do some brands of orange juice, yogurt, margarine and other food products. Plant milk alternatives (such as beverages made from soy, almond, or oats) are often fortified with vitamin D to the amount found in fortified cow's milk (about 100 IU/cup); the Nutrition Facts label will list the actual amount.(Vitamin D — Health Professional Fact Sheet, n.d.)
Both the United States and Canada mandate the fortification of infant formula with vitamin D: 40–100 IU/100 kcal in the United States and 40–80 IU/100 kcal in Canada.”(Vitamin D — Health Professional Fact Sheet, n.d.).
Countries with vitamin D fortification including of dairy
Countries that fortify fluid milk include, Finland, Canada, United States; some dairy products are fortified in Sweden, and Norway.(Itkonen et al., 2018) (See table 1 of referenced paper) Fortification in these countries is due to a mix of Federal, and State or Regional mandatory requirement, or implemented recommendations.
United Kingdom, Ireland, Spain, and Australia have some non-systemic fortification; lack of clear labelling, and understanding of vitamin D, may contribute to lower vitamin D levels on a population basis.
Low COVID-19 in children
Whilst vitamin D levels in children are often low, feed 'formula' is generally always supplemented, thus providing some protection to the very young.(WHO | Vitamin D supplementation and respiratory infections in children, 2019) Toddlers and younger children, probably also on average, get a little more exposure to sunshine that their older peers. Suggestions for Future
Whilst some of the data above, and below, public policy, and population attitudes and knowledge, may provide limited indicative information as to vitamin D deficiency status, ultimately the hypothesis that vitamin D deficiency factors negatively in COVID-19, can only be determined by appropriate testing.
Further, global deficiencies of vitamin D can only be addressed by: encouraging testing, particularly of those in at risk groups, including those in residential institutions; reporting and collation of test result data at national level; maintenance of a rolling annual global database of results; and implementation of National polices for fortification and or supplementation, that appropriately, increase, or decrease, vitamin D intake, based on data, thus minimising risks of population vitamin D deficiencies.
Basal human vitamin D levels
Whilst governments issue advisories, as to optimal vitamin D intakes and blood levels; and there is debate as to optimal intakes and plasma levels; there is only very limited research, in groups with non-westernised lifestyles, to determine natural endogenous human vitamin D levels.
Interestingly, studies in East Africa(Krzyścin et al., 2016; Luxwolda et al., 2012) reported vitamin D levels in non-westernised groups (Av: Masai 119nmol/L; Hadzabe 109nmol/L), were higher than those prescribed by many national advisories.
Caucasian beach guards, in times before significant application of UVB blocking sun creams, have similar levels. Interestingly cattle(Nelson et al., 2016) and swine(Larson-Meyer et al., 2017) raised in the open also have levels in the 40-100ng/l (100–240 nmol/L)(Nelson et al., 2016) range.
Thus endogenous 'natural' levels of vitamin D in humans, livestock and pets, may be significantly higher than those used for national health advisory recommendations. More studies as to human basal endogenous vitamin D levels in non-westernised groups, whilst they still exist, are urgently required; to both to secure better public health; and more objectively define intake guideline requirements.
Policies encouraging supplementation and awareness
Nordic, and other northern European countries, appear to have higher vitamin D levels, despite their more northerly latitude, possibly due to stronger polices on fortification, and encouragement of Vitamin D supplementation in winter. Recent data is scarce, and commercial sales data for vitamin D supplements difficult to find; however food supplementation polices, and attitudes to vitamin D supplementation, as well as limited data; would suggest that vitamin D profiles in northern European countries are likely to be higher, and crucially deficiency less prevalent, in the critical winter season, than in their more southerly neighbours.
For example “In Denmark of participants (n=4,479; 53% females) aged 18–75 years 60 % percent of females and 51% of males were users of supplements”, “total intake of selected micronutrients among the users of dietary supplements of vitamins E and D was increased fourfold and, for iron, selenium, and zinc two- to threefold compared with non-users”(Tetens et al., 2011)
In Norway “According to figures from the Norwegian Prescription Database, the number of users of prescription-only vitamin D3 supplements increased 85-fold in Norway over the six-year period from 2011 to 2017. In the same period, the sales of defined daily doses (20 µg) increased more than a hundredfold. In 2017, sales amounted to 39.5 million defined daily doses. In addition, sales of over-the-counter dietary supplements are high. High-dosage supplements that contain up to 80 µg vitamin D3 per tablet are currently available on the Norwegian market. In contrast, according to the Nordic nutrition recommendations, the recommended daily intake of vitamin D for the general population amounts to 10 µg per day for children and adults and 20 µg per day for those 75 years and older”(Holvik et al., 2019)
In Sweden, vitamin D is a high-profile issue; indeed, a case was brought, in which the Swedish Supreme Administrative Court overruled a local government decision, prohibiting sale of food supplements, including vitamin D, in 2018.(Chu, 2018) A supplement market research agency reports “Vitamin D continued to be the strongest performer with many consumers seeing this as a way to support the development of healthy bones and teeth, particularly as the long dark Swedish winters result in a lack of natural vitamin D from sunlight”.(Vitamins in Sweden | Market Research Report | Euromonitor, n.d.) However, in common with many northern countries, vitamin D insufficiencies remain, more prevalent in patients born outside Europe.(Wändell et al., 2018)
In Finland, vitamin D has long been a central public health issue; indeed, the national recommended intake was at one time 4000IU, and some suggest that type 1 diabetes, and immune related condition, rose in consequence of a subsequent reduction of that recommendation.(Papadimitriou, 2017) According to social media apparently vitamin D is widely supplemented in Finland.(Does the average Finn take vitamin D supplements? : Finland, n.d.)
In Germany and other countries, it may well be that populations are ahead of public policy, in that they are more conscious of the need to supplement with vitamin D. Sadly study data is generally not recent, sometimes 10-20 years old, and thus does not accurately reflect current vitamin D status in populations.
There is clearly significant focus of the importance of vitamin D in Germany as helpfully set out in 'A Critical Appraisal of Strategies to Optimize Vitamin D Status in Germany, a Population with a Western Diet',(Saternus et al., 2019) but clearly also a need for new large-scale data.
German Medical Policy 2.04.135 includes a recommendation as to vitamin D which can be provided free and is recommended for those over 65; “Benefit Application - Consistent with federal mandates, vitamin D supplements are covered as preventive care for individuals age 65 and older(without cost sharing) when the member's contract is subject to those mandates. A written prescription is needed for coverage. The USPSTF recommends exercise or physical therapy and vitamin D supplementation to prevent falls in community-dwelling adults aged 65 years or older who are at increased risk for falls. (Grade B recommendation)”(Testing Serum Vitamin D Level, 2020).
Implementation of national vitamin D recommendations
Most countries have existing health recommendations as to vitamin D intakes, yet significant portions of populations are often deficient and or insufficient. Better data would allow fine tuning of food and direct supplementation polices, to ensure national recommendations were met.
Other dietary factors in oxidative stress related conditions
Iodine, and Omega 3 and 6 lipids and their oxidised products, also have important roles in the interaction of 'body' 'external' membranes, to stimuli, including in regulation of cell junction and immune function, in the lungs, airways, digestive and reproductive organs. Imbalance contributes to oxidative stress, and inflammation episodes including sepsis, and cachexia.(Brown, 2016a) Iodine in the diet is often insufficient; omega 6 linoleic acid is consumed in excess; and Omega 3 linolenic acid is present in diets in insufficient amounts. Other nutrients are also often imbalanced or insufficient, including due to over processing of foods, and changing farming practices.
The paper 'Potential interventions for novel coronavirus in China: A systematic review', highlights the relevance of nutrition to COVID-19 patient treatment, suggesting “the nutritional status of each infected patient should be evaluated before the administration of general treatments” and usefully sets out nutritional factors affecting particular immune pathways, and diseases, in their table 1.(Zhang & Liu, 2020)
Discussion
There is currently a significant apparent difference in the COVID-19 data for, mortality percentage, and number of patients requiring intensive care, between northern European countries and southern European countries, which it is postulated may be related in significant part to vitamin D status, and particularly deficiency.
Whilst it could be suggested that mortality in the northern European countries is yet to emerge, data on infection rate history, and timing in Germany [2] and France(Wikipedia Contributors, 2020) are similar, yet mortality is much higher in France. Time will indeed clarify these issues, but clearly collection of data for studies on the pandemic, is only possible during the active phase of the pandemic, and with the assistance and order of Governments.
Thus, the certainly feasible, and likely issue of vitamin D deficiency, through immune impairment, impacting COVID-19 progression, and mortality, needs raising at Governmental level as a matter of urgency.
Prompt global investigation at depth, using standardised measuring parameters, is required to determine if vitamin D deficiency at population level, factors in; and further, if vitamin D (or its oxidised derivatives) administration alleviates; visible infection rate, severity, and mortality of COVID-19.(Grant et al., 2020)
Given the wide ranging health impacts including in respiratory function, the global nature of vitamin D deficiency, and importance in addressing it;(Roth et al., 2018) good current population data globally on vitamin D status, would be of significant wider public health information value, irrespective of any COVID-19 related outcomes.
Further, it is important for COVID-19 decision making, that mortality rates are; accurate, without “selection bias”, and based on accurate infection rate data; as noted in the article 'A fiasco in the making? As the coronavirus pandemic takes hold, we are making decisions without reliable data.'(Ioannidis, 2020)
Any determination that vitamin D deficiency did moderate risk of; visible infection, progression, and mortality, outcomes of COVID-19; and or that vitamin D derivatives, had clinical uses in reducing the severity of respiratory consequences of COVID-19, as seen in related rodent studies; would have significant policy implications, including for consideration of; seasonal ramifications; advice to remain indoors; and potential benefits of mass vitamin D supplementation in accordance with existing National Governmental Guidelines (with allowance for obesity); and for the reduction of the current consequences of the COVID-19 pandemic, which is having widespread impact on; at risk individuals, public health provision, individual, corporate and national finances, and the global economy.
What constitutes optimal vitamin D levels, including by reference to endogenous levels in non-westernised groups, is an issue for future consideration, as it makes no difference to the need to ensure populations are not at risk of being plain vitamin D deficient.
Conclusions
Acknowledgements
Amrita Sarkar (PhD) was responsible for promotion of the need for, and inclusion of, the graphic illustrations; and helped prepare data, references, content organisation, presentation, and more generally generously contributed time, to preparation of this manuscript. Conflicts of interests
The work is entirely self-funded with no assistance from any external agency or source. The authors have no conflicts of interest.[RB1]
Author contributions
RB and AS wrote the manuscript, organized and supervised the study. Both authors reviewed and approved the manuscript for publication.
References
[RB1]Smaller font is that what you wanted
- dose and time dependent;
- determining factors include - latitude, time of day and year, altitude, albedo reflection from water or snow, UV blocking product use, clothing type, shade, atmospheric pollution levels (UVB can be significantly reduced by city atmospheric pollution); exposure time, and skin type;
- changing life styles mean people are spending less time outdoors, thus are at greater risk of low vitamin D, which may help account for falling levels;
- propensity to skin oxidation, thus burning on exposure to UVB, may be moderated by dietary factors, including Omega 3:6 balance, iodine, and potentially, wider dietary antioxidant capacity derived from plant products for example phenols.(Saric & Sivamani, 2016) Research in these fields is extremely limited, and arguably more is required, to facilitate safer sun exposure.
- Capacity to make vitamin D for any given UVB exposure is significantly impacted by skin tone. Dark skin provides very high UVB absorbance equivalent, depending on shade, equivalent to strong UV skin protection products. Those with dark skins require much greater sun exposure, to make equivalent amounts of vitamin D, to those produced by Caucasians following limited sun exposure. Burning and reddening in Caucasians, following sun exposure, is due to exhaustion of 'antioxidants', including the vitamin D cholesterol derivative precursor, by UVB in sunlight.
- A wide range of polymorphisms exist that will impact many facets of vitamin D creation and metabolism, acquired because they provided greater capacity for survival and reproduction, in given environments.
- Studies suggest that age may diminish capacity to make and store vitamin D. Certainly levels in the elderly tend to be lower.
- Women generally have higher capacity to make UVB, including due to naturally lighter skins than males. The impact of skin lightening on vitamin D production is unknown.
- One of the roles of adipose cells, is to store fat-soluble nutrients, as a reservoir, in a seasonal, weather and food environment. In consequence, logically a portion of available vitamin D is partitioned into the fat compartment. This may account in the obese, for reduced vitamin D plasma availability in comparable populations with the same intake. A study suggests plasma 25OHD3 are closely linked to body fat mass in healthy fasted individuals (interestingly 58 % of the cohort were vitamin D deficient).(Edwards et al., 2020) Obesity / excess weight increases the amount of vitamin D required to maintain adequate vitamin D plasma concentrations. The study, 'The Importance of Body Weight for the Dose Response Relationship of Oral Vitamin D Supplementation and Serum 25-Hydroxyvitamin D in Healthy Volunteers' comments, “We recommend vitamin D supplementation be 2 to 3 times higher for obese subjects and 1.5 times higher for overweight subjects relative to normal weight subjects”.(Ekwaru et al., 2014) Further the review 'Impact of Obesity on Influenza A Virus Pathogenesis, Immune Response, and Evolution' notes, “Obese hosts exhibit delayed and blunted antiviral responses to influenza virus infection, and they experience poor recovery from the disease.”(Honce & Schultz-Cherry, 2019) Those with weight issues are also more at risk of diabetes, a risk factor for COVID-19 severity.
- Showering after sun exposure with soap may reduce Vitamin D by washing it off the skin, however much of the vitamin D is produced by oxidation within the skin and does not appear to be affected by washing.
- The COVID-19 mortality rate in northern European countries, once unaccounted-for COVID-19 infections are added, may prove to be not hugely different from average flu mortality in the USA 2011-2017.
- Vitamin D supplementation in the 'D' deficient increases resistance against influenza.
- Vitamin D likely is a controlling factor in immune pathways, CD38, HLA-DR, CD8 and CD4, already identified in a COVID-19 patient, as key to COVID-19 infection progression and remission.
- Vitamin D can improve ACE related respiratory function, including breathlessness, in animal models.
- There is an urgent need for Governments to facilitate research into the impact of vitamin D in COVID-19, to determine if vitamin D factors in COVID-19, and if vitamin D in its 'passive' or 'active' forms, can be used to treat those with COVID-19 in clinical settings.
- There are no studies on vitamin D and COVID-19. Such studies could be conducted in parallel with COVID-19 testing, and treatment. Costs in the scheme of things would be modest. Rewards could be very significant at many levels.
- If vitamin D deficiency is found to impact COVID-19 outcomes; public health information and supplementation, could reduce COVID-19 severity and mortality, before seasonal effects, risk repeated further COVID-19 pandemic spread, and reoccurrence.
- A Frontline Doctor for the Mail on Sunday, (2020) The look of panic among medics, patients failing to get oxygen in their lungs and dangerous under-dressing of staff: Frontline NHS doctor reveals how coronavirus has unleashed terror in hospital. Daily Mail Published: 22:01 GMT, 21 March 2020 | Updated: 08:13 GMT, 22 March 2020 https://www.dailymail.co.uk/news/article-8138581/Coronavirus-frontline-NHS-doctor-reveals-patients-dying-agony-just-start.html (Accessed 22nd March 2020).
- Annweiler, C., Pochic, S., Fantino, B., Legrand, E., Bataille, R., Montero-Odasso, M., & Beauchet, O. (2010). Serum vitamin D concentration and short-term mortality among geriatric inpatients in acute care settings. Advances in Therapy, 27(4), 245–249. https://doi.org/10.1007/s12325-010-0025-6
- Arnljots, R., Thorn, J., Elm, M., Moore, M., & Sundvall, P. D. (2017). Vitamin D deficiency was common among nursing home residents and associated with dementia: A cross sectional study of 545 Swedish nursing home residents. BMC Geriatrics, 17(1), 229. https://doi.org/10.1186/s12877-017-0622-1
- Begley, S. (2020). New analysis of coronavirus risk: Young adults are not invincible - STAT. https://www.statnews.com/2020/03/18/coronavirus-new-age-analysis-of-risk-confirms-young-adults-not-invincible/ (Accessed 19 Mar 2020).
- Bialek, S., Boundy, E., Bowen, V., Chow, N., Cohn, A., Dowling, N., … Sauber-Schatz, E. (2020). Severe Outcomes Among Patients with Coronavirus Disease 2019 (COVID-19) — United States, February 12–March 16, 2020. MMWR. Morbidity and Mortality Weekly Report, 69(12). https://doi.org/10.15585/mmwr.mm6912e2
- BMJ Preventing a COVID-19 pandemic - Responses. doi: https://doi.org/10.1136/bmj.m810 (Published 28 February 2020) Cite this as: BMJ 2020;368:m810
- Bouillon, R. (2020). Vitamin D status in Africa is worse than in other continents. In The Lancet Global Health (Vol. 8, Issue 1, pp. e20–e21). Elsevier Ltd. https://doi.org/10.1016/S2214-109X(19)30492-9
- Brown, R. A. (2016a). Bioactive oxidised products of omega-6 and omega-3, excess oxidative stress, oxidised dietary intake and antioxidant nutrient deficiencies, in the context of a modern diet. In Omega-3 Fatty Acids: Keys to Nutritional Health (pp. 350–383). Springer International Publishing. https://doi.org/10.1007/978-3-319-40458-5_28
- Brown, R. A. (2016b). The linoleic-to-linolenic dietary intake ratio: The fundamental implications of imbalance and excess looked at from both a functional and an evolutionary perspective: An overview. In Omega-3 Fatty Acids: Keys to Nutritional Health (pp. 321–348). Springer International Publishing. https://doi.org/10.1007/978-3-319-40458-5_27
- Campbell, J. (2020). Vitamin D and immunity - YouTube. YouTube. https://www.youtube.com/watch?v=W5yVGmfivAk (Accessed 16 Mar 2020).
- Cannell, J. J., Vieth, R., Umhau, J. C., Holick, M. F., Grant, W. B., Madronich, S., Garland, C. F., & Giovannucci, E. (2006). Epidemic influenza and vitamin D. In Epidemiology and Infection (Vol. 134, Issue 6, pp. 1129–1140). Cambridge University Press. https://doi.org/10.1017/S0950268806007175.
- Cao, X., Lu, X., Tuo, X. et al. (2019). Angiotensin-converting enzyme 2 regulates endoplasmic reticulum stress and mitochondrial function to preserve skeletal muscle lipid metabolism. Lipids Health Dis 18, 207 (2019). https://doi.org/10.1186/s12944-019-1145-x
- Chen, H., Lu, R., Zhang, Y., & Sun, J. (2018). Vitamin D Receptor Deletion Leads to the Destruction of Tight and Adherens Junctions in Lungs. Tissue Barriers, 6(4), 1–13. https://doi.org/10.1080/21688370.2018.1540904
- Chen, J., Bruce, D., & Cantorna, M. T. (2014). Vitamin D receptor expression controls proliferation of naïve CD8+ T cells and development of CD8 mediated gastrointestinal inflammation. BMC Immunology, 15(1), 6. https://doi.org/10.1186/1471-2172-15-6
- Chu, W. (2018). Supreme Court overrules Sweden's National Food Agency in vitamin D sales ban. NUTRA Ingredients.Com. https://www.nutraingredients.com/Article/2018/11/30/Supreme-Court-overrules-Sweden-s-National-Food-Agency-in-vitamin-D-sales-ban (Accessed 16 Mar 2020).
- Coronavirus Update (Live): 274,171 Cases and 11,354 Deaths from COVID-19 Virus Outbreak - Worldometer. https://www.worldometers.info/coronavirus/ (Accessed 16 Mar 2020).
- Cuomo, A., Maina, G., Bolognesi, S., Rosso, G., Beccarini Crescenzi, B., Zanobini, F., Goracci, A., Facchi, E., Favaretto, E., Baldini, I., Santucci, A., & Fagiolini, A. (2019). Prevalence and Correlates of Vitamin D Deficiency in a Sample of 290 Inpatients With Mental Illness. Frontiers in Psychiatry, 10(MAR), 167. https://doi.org/10.3389/fpsyt.2019.00167
- Disease Burden of Influenza | CDC. Centers for Disease Control and Prevention. https://www.cdc.gov/flu/about/burden/index.html (Accessed 18 Mar 2020).
- Does the average Finn take vitamin D supplements? : Finland. https://www.reddit.com/r/Finland/comments/71h3em/does_the_average_finn_take_vitamin_d_supplements/ (Accessed 16 Mar 2020).
- Dong, Y., Mo, X., Hu, Y., Qi, X., Jiang, F., Jiang, Z., & Tong, S. (2020). Epidemiological Characteristics of 2143 Pediatric Patients With 2019 Coronavirus Disease in China. Pediatrics. https://doi.org/10.1542/peds.2020-0702
- Edwards, M. H., Cole, Z. A., Harvey, N. C., & Cooper, C. (2020). THE GLOBAL EPIDEMIOLOGY OF VITAMIN D STATUS • JARLIFE. The Journal of Aging Research & Lifestyle. http://www.jarlife.net/703-the-global-epidemiology-of-vitamin-d-status.html (Accessed 18 Mar 2020).
- Ekwaru, J. P., Zwicker, J. D., Holick, M. F., Giovannucci, E., & Veugelers, P. J. (2014). The Importance of Body Weight for the Dose Response Relationship of Oral Vitamin D Supplementation and Serum 25-Hydroxyvitamin D in Healthy Volunteers. PLoS ONE, 9(11), e111265. https://doi.org/10.1371/journal.pone.0111265
- Fedson D. (2017) Treating the host response to emerging virus diseases: lessons learned from sepsis, pneumonia, influenza and Ebola Vol 4, No 21 (November 2016) http://atm.amegroups.com/article/view/12396/html
- Galey, P., & Hood, M. (2020, March). Doctors map body's COVID-19 immune response: study. Medical Xpress. https://medicalxpress.com/news/2020-03-doctors-body-covid-immune-response.html (Accessed 18 Mar 2020).
- Grant, W. B., Lahore, H., McDonnell, S. L., Baggerly, C. A., French, C. B., Aliano, J. L., & Bhattoa, H. P. (2020). Vitamin D Supplementation Could Prevent and Treat Influenza, Coronavirus, and Pneumonia Infections. https://doi.org/10.20944/PREPRINTS202003.0235.V1
- Gruber-Bzura, B. M. (2018). Vitamin D and influenza—Prevention or therapy? In International Journal of Molecular Sciences (Vol. 19, Issue 8). MDPI AG. https://doi.org/10.3390/ijms19082419
- Hansdottir, S., & Monick, M. M. (2011). Vitamin D Effects on Lung Immunity and Respiratory Diseases. In Vitamins and Hormones (Vol. 86). https://doi.org/10.1016/B978-0-12-386960-9.00009-5
- Hassan-Smith, Z. K., Jenkinson, C., Smith, D. J., Hernandez, I., Morgan, S. A., Crabtree, N. J., Gittoes, N. J., Keevil, B. G., Stewart, P. M., & Hewison, M. (2017). 25-hydroxyvitamin D3 and 1,25-dihydroxyvitamin D3 exert distinct effects on human skeletal muscle function and gene expression. PLOS ONE, 12(2), e0170665. https://doi.org/10.1371/journal.pone.0170665
- Hewison, M. (2012). An update on vitamin D and human immunity. In Clinical Endocrinology (Vol. 76, Issue 3, pp. 315–325). https://doi.org/10.1111/j.1365-2265.2011.04261.x
- Hidenori Otera1 and Katsuyoshi Mihara Mitochondrial Dynamics: Functional Link with Apoptosis. International Journal of Cell Biology
- Holick, M. F., & Chen, T. C. (2008). Vitamin D deficiency: A worldwide problem with health consequences. American Journal of Clinical Nutrition, 87(4). https://doi.org/10.1093/ajcn/87.4.1080s
- Holvik, K., Meyer, H. E., Madar, A. A., & Brustad, M. (2019). High-dosage vitamin D supplements are unnecessary. Tidsskrift for Den Norske Laegeforening, 139(7). https://doi.org/10.4045/tidsskr.18.0749
- Honce, R., & Schultz-Cherry S. (2019). Impact of Obesity on Influenza A Virus Pathogenesis, Immune Response, and Evolution. Front Immunol. 2019; 10: 1071. doi: 10.3389/fimmu.2019.01071
- Hughes, D. A., & Norton, R. (2009). Vitamin D and respiratory health. In Clinical and Experimental Immunology (Vol. 158, Issue 1, pp. 20–25). John Wiley & Sons, Ltd. https://doi.org/10.1111/j.1365-2249.2009.04001.x
- Hybrigenics presented at the ASH Annual Meeting the potency of inecalcitol to induce CD38 on Multiple Myeloma cell lines. (2017, December). Hybrigenics Pharma, 1–2. http://www.hybrigenics.com/news/articles/getfile/id/524
- Ioannidis, J. P. A. (2020, March). In the coronavirus pandemic, we're making decisions without reliable data. https://www.statnews.com/2020/03/17/a-fiasco-in-the-making-as-the-coronavirus-pandemic-takes-hold-we-are-making-decisions-without-reliable-data/ (Accessed 18 Mar 2020).
- Itkonen, S. T., Erkkola, M., & Lamberg-Allardt, C. J. E. (2018). Vitamin D fortification of fluid milk products and their contribution to vitamin D intake and vitamin D status in observational studies—a review. In Nutrients (Vol. 10, Issue 8). MDPI AG. https://doi.org/10.3390/nu10081054
- Jolliffe, D. A., Greenberg, L., Hooper, R. L., Mathyssen, C., Rafiq, R., De Jongh, R. T., Camargo, C. A., Griffiths, C. J., Janssens, W., & Martineau, A. R. (2019). Vitamin D to prevent exacerbations of COPD: Systematic review and meta-analysis of individual participant data from randomised controlled trials. Thorax, 74(4), 337–345. https://doi.org/10.1136/thoraxjnl-2018-212092
- Kasapidou, E., Oikonomidou, A. C., & Chourdakis, M. (2018). Vitamin D status among Mediterranean regions. Hippokratia, 22(4), 191.
- Kawahito, Y. (2003). The roles of PPARs in immune and inflammatory system: application for arthritic joint disease. Ensho Saisei, 23(2), 74–83. https://doi.org/10.2492/jsir.23.74
- Keane, K. N., Cruzat, V. F., Calton, E. K., Hart, P. H., Soares, M. J., Newsholme, P., & Yovich, J. L. (2017). Molecular actions of Vitamin D in reproductive cell biology. In Reproduction (Vol. 153, Issue 1, pp. R29–R42). BioScientifica Ltd. https://doi.org/10.1530/REP-16-0386
- Ker, H. Le. (2020, March). Hospitals in Germany Ready Themselves for the Worst - DER SPIEGEL. SPIEGEL International. https://www.spiegel.de/international/germany/hospitals-in-germany-ready-themselves-for-the-worst-a-3fe76172-b3f4-4332-9261-561d049b151a (Accessed 21 Mar 2020).
- Kong J, Zhu X, Shi Y, Liu T, Chen Y, Bhan I, Zhao Q, Thadhani R, Li YC. (2013) VDR attenuates acute lung injury by blocking Ang-2-Tie-2 pathway and renin-angiotensin system. Mol Endocrinol. 2013 Dec;27(12):2116-25. doi: 10.1210/me.2013-1146. Epub 2013 Nov 6.
- Krzyścin, J. W., Guzikowski, J., & Rajewska-Wiȩch, B. (2016). Optimal vitamin D3 daily intake of 2000 IU inferred from modeled solar exposure of ancestral humans in Northern Tanzania. Journal of Photochemistry and Photobiology B: Biology, 159, 101–105. https://doi.org/10.1016/j.jphotobiol.2016.03.029
- Larson-Meyer, D. E., Ingold, B. C., Fensterseifer, S. R., Austin, K. J., Wechsler, P. J., Hollis, B. W., Makowski, A. J., & Alexander, B. M. (2017). Sun exposure in pigs increases the vitamin D nutritional quality of pork. PLOS ONE, 12(11), e0187877. https://doi.org/10.1371/journal.pone.0187877
- Lips, P., Cashman, K. D., Lamberg-Allardt, C., Bischoff-Ferrari, H. A., Obermayer-Pietsch, B., Bianchi, M. L., Stepan, J., Fuleihan, G. E.-H., & Bouillon, R. (2019). Current vitamin D status in European and Middle East countries and strategies to prevent vitamin D deficiency: a position statement of the European Calcified Tissue Society in: European Journal of Endocrinology Volume 180 Issue 4 (2019). European Journal of Endocrinology, 180(4), P23–P54.
- Luxwolda, M. F., Kuipers, R. S., Kema, I. P., Janneke Dijck-Brouwer, D. A., & Muskiet, F. A. J. (2012). Traditionally living populations in East Africa have a mean serum 25-hydroxyvitamin D concentration of 115nmol/l. British Journal of Nutrition, 108(9), 1557–1561. https://doi.org/10.1017/S0007114511007161
- Marfey, S. P., Van Meter, R., & Bartlet, M. E. (1975). Interaction between Cholesterol and Calcium Ions in Solution. Zeitschrift Fur Naturforschung - Section C Journal of Biosciences, 30(11–12), 718–721. https://doi.org/10.1515/znc-1975-11-1203
- Martin, T. A., Das, T., Mansel, R. E., & Jiang, W. G. (2007). Enhanced tight junction function in human breast cancer cells by antioxidant, selenium and polyunsaturated lipid. Journal of Cellular Biochemistry, 101(1), 155–166. https://doi.org/10.1002/jcb.21162
- Martin, T. A., Das, T., Shalwitz, R. A., Mansel, R. E., & Jiang, W. G. (2005). Regulation of endothelial tight junctions by selenium, iodine, and its impact on GLA. Cancer Research, 65(9 Supplement).
- Martineau, A. R., Cates, C. J., Urashima, M., Jensen, M., Griffiths, A. P., Nurmatov, U., … Griffiths, C. J. (2016, September 5). Vitamin D for the management of asthma. Cochrane Database of Systematic Reviews. John Wiley and Sons Ltd. https://doi.org/10.1002/14651858.CD011511.pub2
- Martineau, A. R., Jolliffe, D. A., Hooper, R. L., Greenberg, L., Aloia, J. F., Bergman, P., … Camargo, C. A. (2017). Vitamin D supplementation to prevent acute respiratory tract infections: Systematic review and meta-analysis of individual participant data. BMJ (Online), 356. https://doi.org/10.1136/bmj.i6583
- Mawson, A. R. (2013). Role of Fat-Soluble Vitamins A and D in the Pathogenesis of Influenza: A New Perspective. International Scholarly Research Notices, 2013, 1–26. https://doi.org/10.5402/2013/246737
- Monella, L. M., & Priese, A. (2020). Coronavirus: Why does Germany have so few COVID-19 deaths? | Euronews. Euronews. https://www.euronews.com/2020/03/13/coronavirus-why-does-germany-have-so-few-COVID-19-deaths (Accessed 16 Mar 2020).
- Muir, T. (n.d.). Peripheral Blood Mononuclear Cells: A Brief Review | StemExpress. https://www.stemexpress.com/blogs/peripheral-blood-mononuclear-cells/ (Accessed 18 Mar 2020).
- Nelson, C. D., Powell, J. L., Price, D. M., Hersom, M. J., Yelich, J. V., Drewnoski, M. E., Bird, S. L., & Bridges, G. A. (2016). Assessment of serum 25-hydroxyvitamin D concentrations of beef cows and calves across seasons and geographical locations1. Journal of Animal Science, 94(9), 3958–3965. https://doi.org/10.2527/jas.2016-0611
- Nelson, Corwin D., Lippolis, J. D., Reinhardt, T. A., Sacco, R. E., Powell, J. L., Drewnoski, M. E., O'Neil, M., Beitz, D. C., & Weiss, W. P. (2016). Vitamin D status of dairy cattle: Outcomes of current practices in the dairy industry. Journal of Dairy Science, 99(12), 10150–10160. https://doi.org/10.3168/jds.2016-11727
- Nwosu, B. U., Maranda, L., Berry, R., Colocino, B., Flores, C. D., Folkman, K., Groblewski, T., & Ruze, P. (2014). The vitamin D status of prison inmates. PLoS ONE, 9(3), e90623. https://doi.org/10.1371/journal.pone.0090623
- Papadimitriou, D. T. (2017). The big Vitamin D mistake. In Journal of Preventive Medicine and Public Health (Vol. 50, Issue 4, pp. 278–281). Korean Society for Preventive Medicine. https://doi.org/10.3961/jpmph.16.111
- Parhami, F., Morrow, A. D., Balucan, J., Leitinger, N., Watson, A. D., Tintut, Y., Berliner, J. A., & Demer, L. L. (1997). Lipid oxidation products have opposite effects on calcifying vascular cell and bone cell differentiation. A possible explanation for the paradox of arterial calcification in osteoporotic patients. Arteriosclerosis, Thrombosis, and Vascular Biology, 17(4), 680–687. https://doi.org/10.1161/01.atv.17.4.680
- Park, J. H., Hong, I. Y., Chung, J. W., & Choi, H. S. (2018). Vitamin D status in South Korean population. Medicine (United States), 97(26). https://doi.org/10.1097/MD.0000000000011032
- Peláez, V. C., Ausín L., Mambrilla, M. R., Gonzalez-Sagrado M., Pérez J.L. Castrillón, P. (2017) Severe Vitamin D Deficiency, Functional Impairment And Mortality In Elderly Nursing Home Residents. Jarlife Aorik-23 2021 Boston, MA, USA,
- Pike, J. W., Meyer, M. B., Benkusky, N. A., Lee, S. M., St. John, H., Carlson, A., Onal, M., & Shamsuzzaman, S. (2016). Genomic Determinants of Vitamin D-Regulated Gene Expression. In Vitamins and Hormones (Vol. 100, pp. 21–44). Academic Press Inc. https://doi.org/10.1016/bs.vh.2015.10.011
- Portela, M. L.,, Mónico, A., Barahona, A., Dupraz, H., Sol Gonzales-Chaves, M. M., Zeni, S. N. (2010) Comparative 25-OH-vitamin D level in institutionalized women older than 65 years from two cities in Spain and Argentina having a similar solar radiation index. Nutrition 26(3):283-9 DOI: 10.1016/j.nut.2009.04.022
- Prisoners have very low vitamin D and get TB, influenza, and depression. https://vitamindwiki.com/Prisoners+have+very+low+vitamin+D+and+get+TB%2C+influenza%2C+and+depression (Accessed 16 Mar 2020).
- Ricca, C., Aillon, A., Bergandi, L., Alotto, D., Castagnoli, C., & Silvagno, F. Vitamin D Receptor Is Necessary for Mitochondrial Function and Cell Health. Int J Mol Sci. 2018 Jun; 19(6): 1672. doi: 10.3390/ijms19061672
- Roth, D. E., Abrams, S. A., Aloia, J., Bergeron, G., Bourassa, M. W., Brown, K. H., Calvo, M. S., … & Whiting, S. J. (2018). Global prevalence and disease burden of vitamin D deficiency: a roadmap for action in low- and middle-income countries. Annals of the New York Academy of Sciences, 1430(1), 44–79. https://doi.org/10.1111/nyas.13968.
- Santos, G. C., Zeidler, J. D., Pérez-Valencia, J. A., Sant'Anna-Silva, A. C. B., Da Poian, A. T., El-Bacha, T., Almeida, F. C. L. (2017) Metabolomic analysis reveals vitamin D-induced decrease in polyol pathway and subtle modulation of glycolysis in HEK293T cells. Sci Rep 7:9510.
- Saric, S., & Sivamani, R. K. (2016). Polyphenols and sunburn. In International Journal of Molecular Sciences (Vol. 17, Issue 9). MDPI AG. https://doi.org/10.3390/ijms17091521
- Saternus, R., Vogt, T., & Reichrath, J. (2019). A critical appraisal of strategies to optimize vitamin d status in germany, a population with a western diet. Nutrients, 11(11). https://doi.org/10.3390/nu11112682
- Sertznig, P., Dunlop, T., Seifert, M., Tilgen, W., & Reichrath, J. (2009). Cross-talk between vitamin D receptor (VDR)- and peroxisome proliferator-activated receptor (PPAR)-signaling in melanoma cells. Anticancer Research, 29(9), 3647–3658.
- Sims, A. C., Tilton, S. C., Menachery, V. D., Gralinski, L. E., Schafer, A., Matzke, M. M., … & Baric, R. S. (2013). Release of Severe Acute Respiratory Syndrome Coronavirus Nuclear Import Block Enhances Host Transcription in Human Lung Cells. Journal of Virology, 87(7), 3885–3902. https://doi.org/10.1128/jvi.02520-12
- Spiro, A., Buttriss, J, L. (2014) Vitamin D: An overview of vitamin D status and intake in Europe. Nutr Bull. Dec;39(4):322-350. DOI: 10.1111/nbu.12108
- Testing Serum Vitamin D Level. (2020). MEDICAL POLICY –2.04.135 Primera Blue Cross https://www.premera.com/medicalpolicies/2.04.135.pdf (Accessed 20th March 2020)
- Tetens, I., Biltoft-Jensen, A., Spagner, C., Christensen, T., Gille, M. B., Bügel, S., & Rasmussen, L. B. (2011). Intake of micronutrients among Danish adult users and non-users of dietary supplements. Food and Nutrition Research, 55. https://doi.org/10.3402/fnr.v55i0.7153
- Thevarajan, I., Nguyen, T. H. O., Koutsakos, M., Druce, J., Caly, L., van de Sandt, C. E., Jia, X., Nicholson, S., Catton, M., Cowie, B., Tong, S. Y. C., Lewin, S. R., & Kedzierska, K. (2020). Breadth of concomitant immune responses prior to patient recovery: a case report of non-severe COVID-19. Nature Medicine, 1–3. https://doi.org/10.1038/s41591-020-0819-2
- Tintut, Y., Hsu, J. J., & Demer, L. L. (2018). Lipoproteins in Cardiovascular Calcification: Potential Targets and Challenges. In Frontiers in Cardiovascular Medicine (Vol. 5, p. 172). Frontiers Media S.A. https://doi.org/10.3389/fcvm.2018.00172
- Van Beusekom, M. (2020, March 18). US studies offer clues to COVID-19 swift spread, severity | CIDRAP. Retrieved March 23, 2020, from http://www.cidrap.umn.edu/news-perspective/2020/03/us-studies-offer-clues-COVID-19-swift-spread-severity
- Vitamin D — Health Professional Fact Sheet. National Institutes of Health US Deparment of Health & Human Services https://ods.od.nih.gov/factsheets/VitaminD-HealthProfessional/ (Accessed 20th March 2020)
- Vitamins in Sweden | Market Research Report | Euromonitor. https://www.euromonitor.com/vitamins-in-sweden/report (Accessed 16 Mar 2020).
- Vringer, E., Tait, S. W. G., Mitochondria and Inflammation: Cell Death Heats UpFront. Cell Dev. Biol., 27 June 2019 | https://doi.org/10.3389/fcell.2019.00100
- Vukić, M., Neme, A., Seuter, S., Saksa, N., de Mello, V. D. F., Nurmi, T., Uusitupa, M., Tuomainen, T.-P., Virtanen, J. K., & Carlberg, C. (2015). Relevance of Vitamin D Receptor Target Genes for Monitoring the Vitamin D Responsiveness of Primary Human Cells. PLOS ONE, 10(4), e0124339. https://doi.org/10.1371/journal.pone.0124339
- Walsh, J., Bowles S., & Evans A. (2011) Vitamin D in obesity. Curr Opin Endocrinol Diabetes Obes. 2017 Dec;24(6):389-394. doi: 10.1097/MED.0000000000000371.
- Wändell, P., Ayoob, S., Mossberg, L., & Andreasson, A. (2018). Vitamin D deficiency was common in all patients at a Swedish primary care centre, but more so in patients born outside of Europe. Journal of Public Health (Germany), 26(6), 649–652. https://doi.org/10.1007/s10389-018-0910-z
- Wang, Z., Wang, Y., Xu, B., Liu, J., Ren, Y., Dai, Z., Cui, D., Su, X., Si, S., & Song, S. J. (2017). Vitamin D improves immune function in immunosuppressant mice induced by glucocorticoid. Biomedical Reports, 6(1), 120–124. https://doi.org/10.3892/br.2016.817
- Watkins, J. (2020). Preventing a COVID-19 pandemic. BMJ, 368, m810. https://doi.org/10.1136/bmj.m810
- Wei, J., Zhu, A., & Ji, J. S. (2019). A Comparison Study of Vitamin D Deficiency among Older Adults in China and the United States. Scientific Reports, 9(1), 1–11. https://doi.org/10.1038/s41598-019-56297-y
- WHO | Vitamin D supplementation and respiratory infections in children. (2019). WHO. https://www.who.int/elena/titles/vitamind_pneumonia_children/en/ (Accessed 20th March 2020)
- WHO Coronavirus disease (COVID-2019) situation reports. Situation report - 61 https://www.who.int/docs/default-source/coronaviruse/situation-reports/20200321-sitrep-61-COVID-19.pdf?sfvrsn=6aa18912_2 (Accessed 21st March 2020)
- Wikipedia Contributors. (2020a). coronavirus pandemic in France - Wikipedia. Wikipedia, Free Encycl. https://en.wikipedia.org/w/index.php?title=2020_coronavirus_pandemic_in_France&oldid=946766885 (Accessed 22nd March 2020)
- Wikipedia contributors. (2020b) Coronavirus Pandemic in Germany Wikipedia. Wikipedia, Free Encycl. https://en.wikipedia.org/w/index.php?title=2020_coronavirus_pandemic_in_Germany&oldid=946714813 (Accessed 21st March 2020). Coronavirus disease 2019 (COVID-19)Situation Report –60
- Wikipedia Contributors. (2020c) coronavirus pandemic in Italy Wikipedia. Wikipedia, Free Encycl. https://en.wikipedia.org/w/index.php?title=2020_coronavirus_pandemic_in_Italy&oldid=946699884 (Accessed 21st March 2020)
- Wikipedia contributors. (2020d). coronavirus pandemic in Sweden - Wikipedia. Wikipedia, Free Encycl. https://en.wikipedia.org/w/index.php?title=2020_coronavirus_pandemic_in_Sweden&oldid=946766953 (Accessed 22nd March 2020)
- Wimalawansa S. (2019) Vitamin D deficiency: effects on oxidative stress, epigenetics, gene regulation, and aging biology 2019, 8, 30; doi:10.3390/biology8020030
- Xu, J., Jian, Y., Chen, J,. Luo, Q., Zhang, Q., Zhang, H. (2017) Vitamin D alleviates lipopolysaccharide‑induced acute lung injury via regulation of the renin‑angiotensin system Molecular Medicine Reports, Volume 16 Issue 5 Print ISSN: 1791-2997 Online ISSN:1791-3004 https://doi.org/10.3892/mmr.2017.7546
- Wu, Y.-C., Chen, C.-S., & Chan, Y.-J. (2020). The outbreak of COVID-19. An overview. Journal of the Chinese Medical Association, 1. https://doi.org/10.1097/jcma.0000000000000270
- Yang, J,. Zhang, H., Xu, Z. (2016) Effect of Vitamin D on ACE2 and Vitamin D receptor expression in rats with LPS-induced acute lung injury. DOI: 10.3760/cma.j.issn.1671-0282.2016.12.016
- Zhang, L., & Liu, Y. (2020). Potential Interventions for Novel Coronavirus in China: A Systemic Review. Journal of Medical Virology. https://doi.org/10.1002/jmv.25707
- Zhang, Y., Wu, S., & Sun, J. (2013). Vitamin D, vitamin D receptor and tissue barriers. Tissue Barriers, 1(1), e23118. https://doi.org/10.4161/tisb.23118